Glioblastoma (GBM), is one of the most aggressive types of cancer that can occur in the brain or spinal cord. This cancer has among the highest mortality rates for primary brain tumors. Most diagnoses last for 11-15 months, with few reports of patients surviving past 30 months of diagnosis. While there have been amazing medical advances made over the last 100 years in western society, there have been no significant advances for improving the survival rate of glioblastoma. Today, this disease remains largely unmanageable.
This review article by Seyfried et al. gives an in depth analysis of what metabolic factors may drive glioblastoma cell growth including fermentation metabolism, blood glucose, glutamine, chemotherapy, and more. Additionally, this review goes through potential factors and therapies for attenuating glioblastoma cell growth. These factors include ketone bodies, reducing blood glucose levels, and simultaneous restriction of glutamine and blood glucose levels. Seyfried and colleagues state that the potential cause for such delay in advancements with treating glioblastoma is likely due to the inability to recognize this cancer as a metabolic disorder. What interests us at Ketonutrition, and likely many of the individuals of our audience, is the role nutrition and ketones may play in the metabolic dysfunctions of glioblastoma. The authors of this article elegantly lay out the holes in current therapies and how we might want to shape future research to fill in the gaps of current treatment.
Drivers of Glioblastoma Cell Growth
Fermentation metabolism is defined as the extraction of energy from carbohydrates through the absence of oxygen. While fermentation metabolism produces less ATP per molecule of glucose than oxidative metabolism, it does so rapidly. Most cancers, especially glioblastoma, depend on fermentation metabolism to create ATP. The abnormalities of mitochondrial structure and function in GBM drive this cancer’s dependency for fermentation metabolism. Additionally, the presence of glucose and glutamine also increase fermentation and further drive glioblastoma cell growth.Waste products of fermentation include lactic acid, glutamic acid, and succinic acid which acidify the microenvironment of GBM and acidification of the microenvironment is largely responsible for the drug resistance, enhanced invasion, immunosuppression, and metastasis in GBM patients.
Mitochondrial substrate level phosphorylation (mSLP) drives ATP synthesis and microenvironment acidification of GBM. The glutamine nitrogen produced from glutaminolysis is essential for creating nucleotides and amino acids. However, the waste products of glutaminolysis (primarily glutamic acid and succinic acid) also contribute to the acidification of the GBM microenvironment (1, 2, 3, 4).
Current treatment for GBM patients include debulking surgery, radiation, chemotherapy, and steroids. However, these treatments aside from surgical debulking may also further acidify the GBM microenvironment and drive GBM growth (5, 6, 7). Radiotherapy disrupts glutamine-glutamate cycle in neural tissue, which increases levels of glutamine and glutamic acid. Glutamic acid is an excitotoxic amino acid that further increases GBM invasion. Radiotherapy damages the brain microenvironment, which causes increases in glucose and glutamine availability to tumor cells, further increasing tumor growth through hyperglycolysis, necrosis, and acidification.
There is evidence to show that survival is lower in GBM patients with higher blood glucose levels than in patients with lower blood glucose levels (5, 8). Oftentimes, steroids are prescribed alongside standard treatments to reduce vasogenic edema which results from disruption of the blood-brain barrier allowing protein-rich fluid to accumulate in extracellular space. These steroids increase blood glucose levels which further drive tumor growth. Radiotherapy also increases blood glucose levels and produces highly invasive metastatic cells in GBM patients (5, 9).
Lactic acid, an end product of glycolysis which increases GBM microenvironment acidity and tumor occurrence. Therefore, dietary restriction of blood glucose levels may also decrease microenvironment acidification through decreasing inflammation, angiogenesis, and increasing tumor cell apoptosis.
Reducing Acidification of the Glioblastoma Microenvironment
Metabolic therapy involves diet and drug combinations that target the availability of glucose and glutamine while also elevating non-fermentable, anti-inflammatory, ketone bodies (10). Due to GBM’s inability to use ketone bodies to produce ATP, ensuring that ketone levels are elevated may play an integral role in suppressing GBM tumor cell growth (11). A ketogenic diet and water only fasting both decrease blood glucose levels while increasing D-Beta hydroxybutyrate levels. Additionally, decreasing blood glucose levels decrease insulin-like growth factor 1, which is a known driver of tumor growth. Transitioning patients to ensure that they are functioning on a ketone driven metabolism will decrease circulating glucose levels, thus decreasing extracellular acidification from lactic acid production. Reducing availability of glucose also increases metabolic stress on all neoplastic GBM cells that are dependent on glycolysis for growth (12).
The simultaneous restriction of glutamine and glucose alongside a ketogenic diet may reduce GBM growth and acidification. Studies have shown that glutamine drives GBM growth (9). Seyfried et al. showed that a glutamine-targeting analogue with a calorie restricted ketogenic diet decreases tumor growth and increases survival in preclinical models of GBM (figure 1).
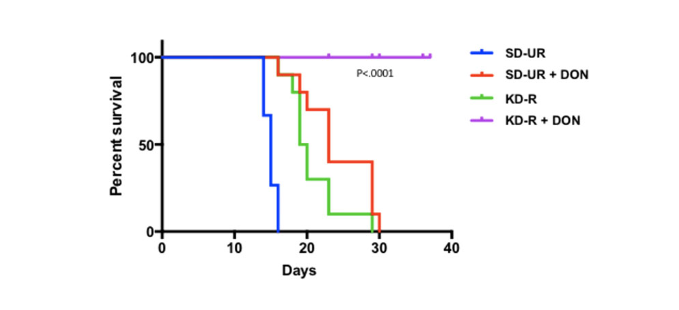
Figure 1. Influence of diet/drug therapy on overall survival of VM/Dk mice with the VM-M3 invasive GBM. Calorie restricted ketogenic diet (KD-R), 6-diazo-5-oxo-L-norleucine (DON), standard high-carbohydrate diet (SD-UR). Source: Mukherjee P et al. Therapeutic benefit of combining calorie-restricted ketogenic diet and glutamine targeting in late-stage experimental glioblastoma. Commun Biol (2019) 2:200. doi: 10.1038/s42003-019-0455-x
The addition of the ketogenic diet in suppressing glutamine levels facilitates delivery of this analogue and other small molecules through the blood brain barrier (10). Additionally, hyperbaric oxygen therapy alongside therapeutic ketosis has also shown to decrease angiogenesis and inflammation of the microenvironment (12). Amazingly, one study has documented the long term survival of a GBM patient who has survived the diagnosis for 8 years so far (13). This is likely attributed to a combination of the patient’s young age, a low carb ketogenic diet, 1DH1 mutation, and avoidance of radiation and steroids for treatment. This study highlights the IDH1 mutation as a potential therapeutic drug that targets the glycolysis and glutaminolysis pathways.
Seyfried et al. ‘s conclusion can be concisely summarized by the following statement: “The greater the availability of fermentable fuels, the greater is the resistance to therapy.” Therefore, this review creates a trajectory for more scientists and medical professionals to seek therapeutic strategies that restrict fermentation while increasing levels of non-fermentable ketone bodies to reduce acidification, eliminate neoplastic tumor cells, and as a result improve GBM management.
Written by Karina Noboa
References:
- Chinopoulos C, Seyfried TN. Mitochondrial substrate-level phosphorylation as energy source for glioblastoma: Review and hypothesis. ASN Neuro (2018) 10:1759091418818261. doi: 10.1177/1759091418818261
- Seyfried TN, Arismendi-Morillo G, Mukherjee P, Chinopoulos C. On the origin of atp synthesis in cancer. iScience (2020) 23(11):101761. doi: 10.1016/ j.isci.2020.101761
- Tannahill GM, Curtis AM, Adamik J, Palsson-McDermott EM, McGettrick AF, Goel G, et al. Succinate is an inflammatory signal that induces IL-1beta through HIF-1alpha. Nature (2013) 496(7444):238–42. doi: 10.1038/nature11986
- Slaughter AL, D’Alessandro A, Moore EE, Banerjee A, Silliman CC, Hansen KC, et al. Glutamine metabolism drives succinate accumulation in plasma and the lung during hemorrhagic shock. J Trauma Acute Care Surg (2016) 81(6):1012–9. doi: 10.1097/TA.0000000000001256
- Seyfried TN, Shelton L, Arismendi-Morillo G, Kalamian M, Elsakka A, Maroon J, et al. Provocative question: Should ketogenic metabolic therapy become the standard of care for glioblastoma? Neurochem Res (2019) 44 (10):2392–404. doi: 10.1007/s11064-019-02795-4
- Ahmadloo N, Kani AA, Mohammadianpanah M, Nasrolahi H, Omidvari S, Mosalaei A, et al. Treatment outcome and prognostic factors of adult glioblastoma multiforme. J Egypt Natl Canc Inst (2013) 25(1):21–30. doi: 10.1016/ j.jnci.2012.11.001
- Stensjoen AL, Solheim O, Kvistad KA, Haberg AK, Salvesen O, Berntsen EM. Growth dynamics of untreated glioblastomas in vivo. Neuro Oncol (2015) 17 (10):1402–11. doi: 10.1093/neuonc/nov029
- Derr RL,YeX,IslasMU ,Desideri S ,Saudek CD,Grossman ‘S.Association between hyperglycemia and survival in patients with newly diagnosed glioblastoma. J Clin Oncol (2009) 27(7):1082–6. doi: 10.1200/JCO.2008.19.1098
- Seyfried, T. N., Arismendi-Morillo, G., Zuccoli, G., Lee, D. C., Duraj, T., Elsakka, A. M., & Chinopoulos, C. (2022). Metabolic management of microenvironment acidity in glioblastoma. Frontiers in Oncology, 4051.
- Mukherjee P, Augur ZM, Li M, Hill C, Greenwood B, Domin MA, et al. Therapeutic benefit of combining calorie-restricted ketogenic diet and glutamine targeting in late-stage experimental glioblastoma. Commun Biol (2019) 2:200. doi: 10.1038/s42003-019-0455-x
- Zhou W, Mukherjee P, Kiebish MA, Markis WT, Mantis JG, Seyfried TN. The calorically restricted ketogenic diet, an effective alternative therapy for malignant brain cancer. Nutr Metab (Lond) (2007) 4:5. doi: 10.1186/1743-7075- 4-5
- Seyfried TN, Yu G, Maroon JC, D’Agostino DP. Press-pulse: a novel therapeutic strategy for the metabolic management of cancer. Nutr Metab (Lond) (2017) 14:19. doi: 10.1186/s12986-017-0178-2
- Seyfried TN, Shivane AG, Kalamian M, Maroon JC, Mukherjee P, Zuccoli G. Ketogenic metabolic therapy, without chemo or radiation, for the long-term management of idh1-mutant glioblastoma: An 80-month follow-up case report. Front Nutr (2021) 8:682243. doi: 10.3389/fnut.2021.682243